Environmental Transformations and Interactions
Creating Synthetic Habitats for Soil Ecology Research
EMSL capabilities help predict biogeochemical interactions that could mitigate climate change
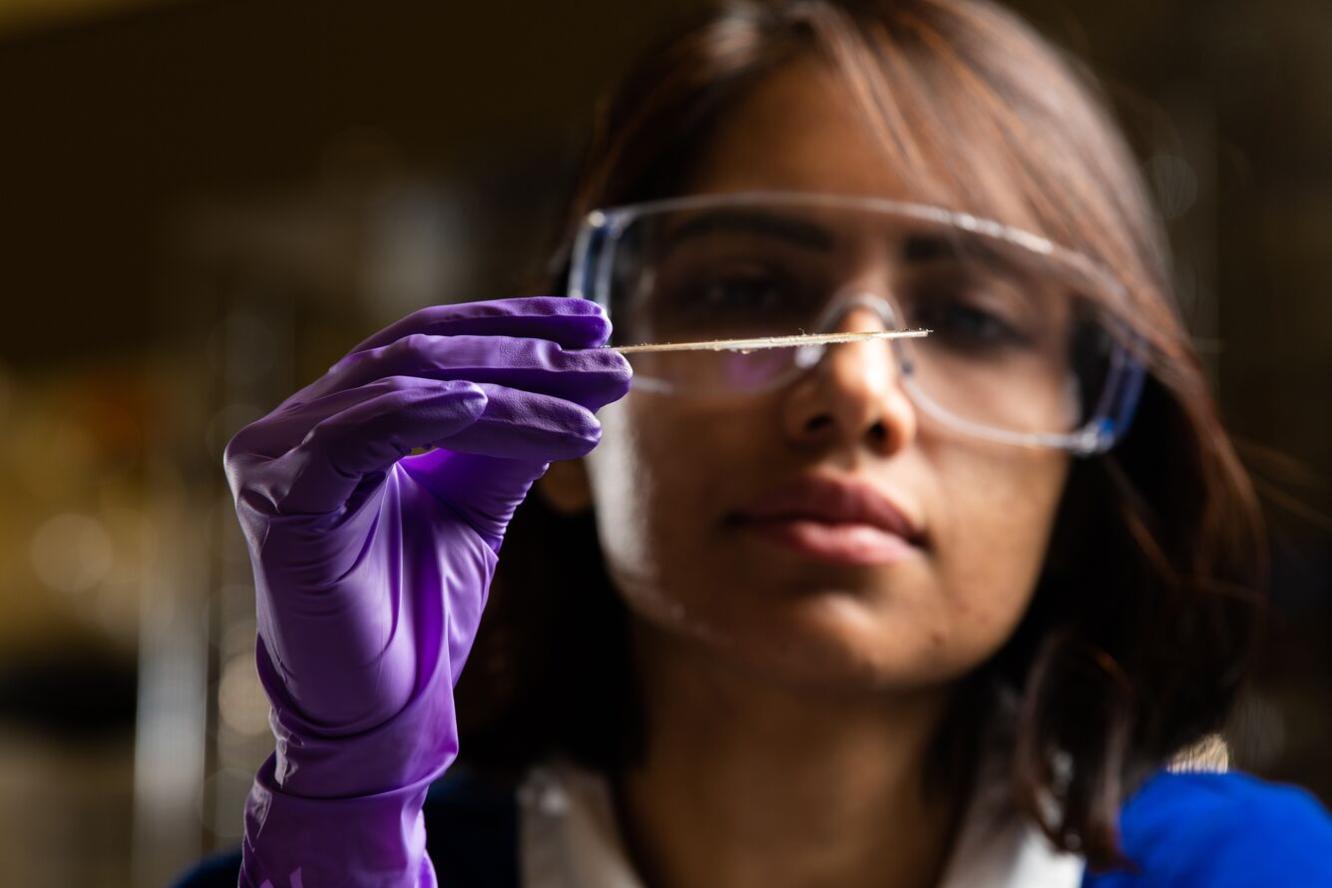
At EMSL, chemist Arunima Bhattacharjee uses synthetic materials to create soil-like microenvironments for studying microbe–mineral interactions. (Photo by Andrea Starr | Pacific Northwest National Laboratory)
Below the Earth’s surface, there are natural pathways, known as biogeochemical cycles, that constantly circulate chemical elements in an ecosystem.
These biogeochemical cycles can’t function without communities of soil microorganisms, also called soil microbiomes. These microbiomes affect carbon cycling, including climate change and a host of other vital ecological processes. However, soil is highly complex and spatially varies in composition, which makes it difficult to study in its natural state. This complexity presents significant challenges for spatial visualization as well as precise measurements of microbial and plant community dynamics in soil.
To overcome these challenges, researchers at the Environmental Molecular Sciences Laboratory (EMSL), a Department of Energy user facility, developed an epoxy-glass-based microfluidics technology that enables multimodal on-chip imaging of microbial and plant community dynamics.
“These synthetic soil habitats can be developed in any design format proposed by a user based on their specific research questions,” said EMSL chemist Arunima Bhattacharjee. These habitats enable on-chip imaging using a variety of EMSL instrumentation.
The capabilities work in concert with EMSL’s chemical imaging and mass spectrometry instrumentation and multi-omics technologies. All are available to researchers through the EMSL User Program.
Bhattacharjee and Pauling Fellow Jayde Aufrecht will provide potential users with information on how to access and apply these habitats and technologies for soil ecology research at a free webinar at noon on April 27.
Mineral microfluidics
EMSL’s novel mineral microfluidics technology incorporates solid-phase soil minerals into microfluidic chips to capture sentinel elements of the soil microbial habitat. Through an etching process, these minerals are brought to the surface of the chip, providing an interface for investigating mineral–microbe interactions.
“This technology allows a user to create mineral hotspots on-chip and to study mineral weathering and uptake of inorganic nutrients by soil microorganisms. I have used these mineral microfluidics chips to study fungal-induced weathering of K-feldspars and K uptake mechanisms by fungi,” said Bhattacharjee.
These devices have a highly flexible microfluidics design and allow researchers to study soil, plants, and root systems in a controlled environment through multimodal on-chip imaging.
Users with a successful user proposal can contact Bhattacharjee for implementing the mineral microfluidics and epoxy microfluidics technologies into their device design of choice.
RhizoChip
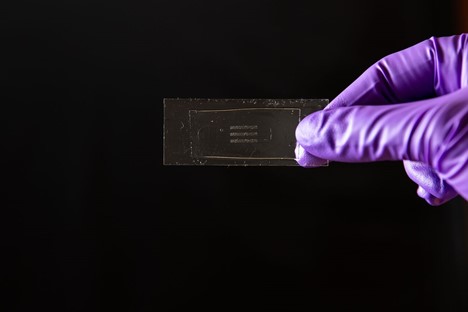
A synthetic rhizosphere, known as Rhizosphere-on-a-Chip, or RhizoChip, is among the resources available at EMSL. RhizoChip allows researchers to simulate and visualize underground interactions between soil, plants, and their related microbiomes over time.
This transparent soil habitat was created by researchers from Pacific Northwest National Laboratory and Oak Ridge National Laboratory using microfluidics technology. RhizoChip provides a microscale soil environment that retains the physical structure of natural soils and can be used to map the molecular environment of roots. This capability can be paired with EMSL’s matrix-assisted laser desorption/ionization (MALDI) mass spectrometer and nanoscale secondary ion mass spectrometry capability for spatial metabolomics research.
EMSL offers users the ability to combine the holistic chip with EMSL’s in-house chemical imaging and metabolomics work. Once a user proposal is accepted, researchers send seeds to Aufrecht, who will grow the plant for up to a month and return experimental data to the user. EMSL can also send the RhizoChip to the user’s home institution.
Aufrecht and a team of researchers recently used the RhizoChip to grow a model grass species, Brachypodium distachyon, and to visualize the development of roots through the synthetic habitat. The research was aimed at understanding how photosynthesized carbon is exuded into the rhizosphere.
The team experimentally mapped carbon hotspots in the rhizosphere by attaching the RhizoChip to a permeable membrane and applied a mass spectrometry technique to validate the presence of hotspots. The researchers found that amino acids are not exuded uniformly along the roots.
“With the RhizoChip, we can recreate hotspots in the rhizosphere,” Aufrecht said. “Then we can add microbes to the plant to see how microbiomes might co-localize with the exudate hotspots.”
Bioprinting
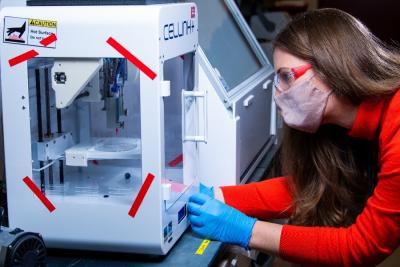
Also available through EMSL is bioprinting—a technology that uses biocompatible hydrogels to create living 3D structures to act as microbial habitats. Like RhizoChip, bioprinting simulates underground environments and provides researchers with opportunities to measure biogeochemical interactions.
EMSL staff have used bioprinting to print bacteria and fungi directly into synthetic soil aggregates, which are a functional unit of the soil ecosystem. This capability replicates the heterogeneous pore space of natural soil aggregates and can be modified with cells, minerals, organic matter, or microplastics to simulate a realistic chemical environment. The synthetic soil habitats can be printed in a 96-well plate format and processed for high-throughput phenotyping of spatially organized microbial communities.
Users can specify which microbes should be printed, which compounds to add to the print, and which 3D print design to use, Aufrecht said.
By pairing the bioprinted soils with MPLEx, a single step extraction method, researchers can recover detectable metabolites, lipids, and proteins from a single synthetic aggregate—a feat that is not currently possible with natural soil aggregates. “Bioprinting synthetic soil aggregates is an alternative way of studying microbial hot spots in the soil,” Aufrecht said. “Synthetic soil aggregates are highly tunable. You can mix in different solid-phase compounds, you can image the microbial communities within the aggregates, and you watch them restructure their habitat. And you can plug them into EMSL’s multi-omics pipeline to get metabolomics, lipidomics, proteomics, and genomics data from a single bioprinted aggregate.”
Learn more about these capabilities on April 27 at the EMSL LEARN Webinar Series presentation, Using Synthetic Habitats for Soil Ecology Research.