Drawing Inspiration from the Muscle of the Mussel Foot
Scientists aim to mimic a mussel’s ability to stick to surfaces to bioengineer novel materials
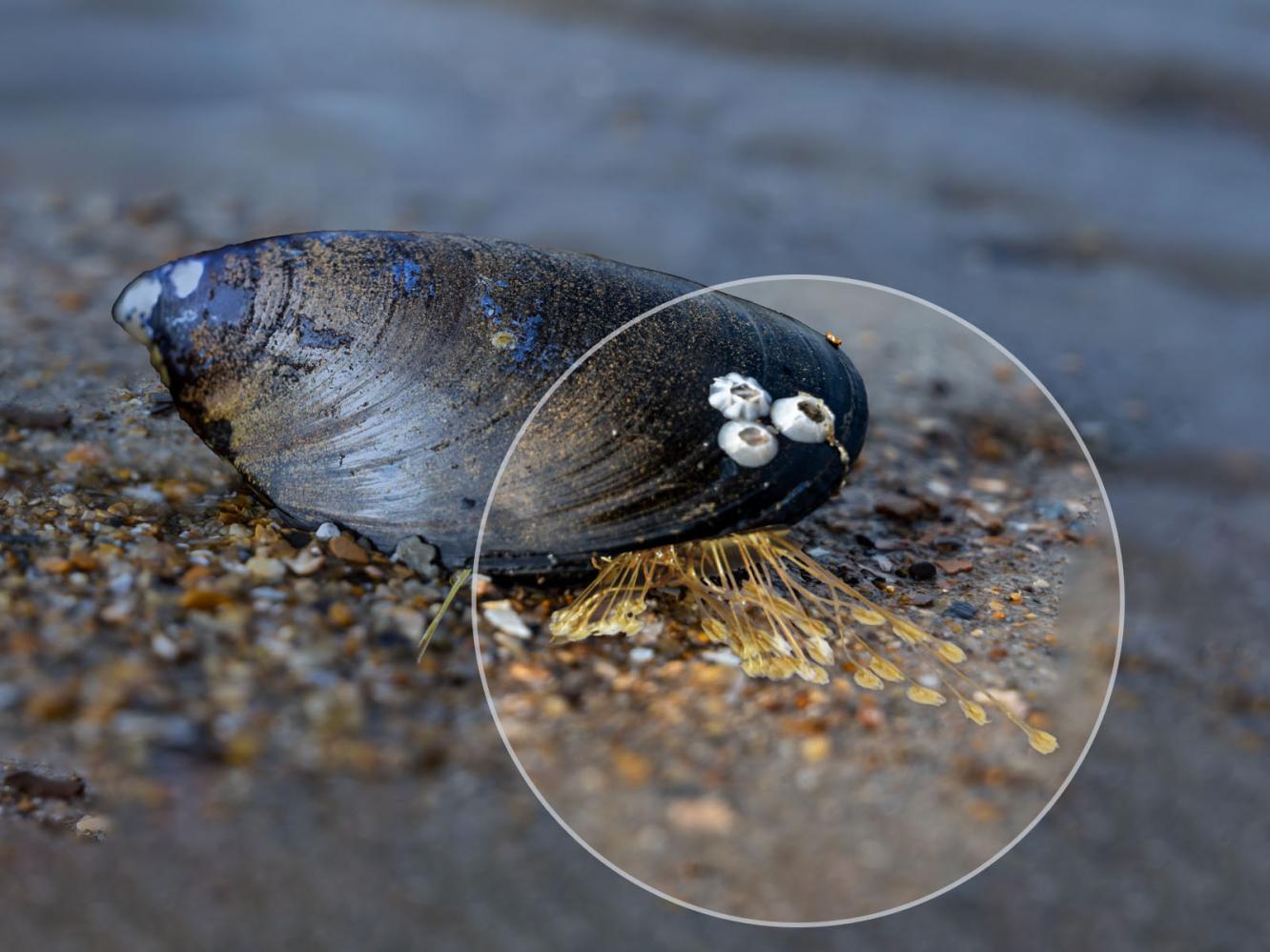
A research team is developing a hydrogel based on the adhesive properties of mussel foot proteins that could seal cracks in boats and act as a replacement for surgical sutures. (Image by Nathan Johnson | Pacific Northwest National Laboratory)
In the vastness of the world’s oceans, the mussel—a mere two to six inches in stature—is proving that strength comes in all sizes.
Specifically, this brawn comes from an even more unlikely part of the mussel—its “foot,” which is the largest organ of these bivalve creatures. The mussel uses its foot, or byssus, to move, to defend itself, and to burrow through sand, silt, and gravel. The muscular foot secretes proteins with powerful adhesive-like fibers, known as the “beard,” which stick to rocks, piers, and even other mussels, making it a longstanding, enviable creator of natural adhesive.
For more than half a century, scientists have been studying mussel foot proteins and how to synthetically duplicate their natural strength. In more recent years, researchers have begun exploring the creation of synthetic versions of mussel foot proteins with potential industrial and medical applications. Research using synthetic biology has demonstrated that recombinant proteins, composed of hybrid mussel foot and spider silk peptide segments, could be used as a biodesign for novel hydrogels with applications as underwater adhesives.
A team, led by Marcus Foston of Washington University in St. Louis, is using advanced capabilities at the Environmental Molecular Sciences Laboratory (EMSL), a Department of Energy user facility, to study material generated using biology, specifically examining how a protein’s amino acid sequence can affect hydrogel composition, structure, and performance. The long-term goal of this project: to develop hydrogels that could seal the cracks in boats or as a surgical adhesive.
Foston’s project is one of 20 that were awarded funding through an EMSL Exploratory Research call, which enables access to the EMSL user facility’s scientific instrumentation and expertise to advance research in environmental and biological science. Listen to Foston explain his research to develop this hydrogel on the Bonding Over Science podcast episode, "The Muscle of Mussel."
The history of studying mussel foot proteins
Within the scientific community, the sticky allure of the mussel foot proteins has led to decades of study around adhesion mechanisms, the design of biosynthetic proteins, and the enhancement of an amino acid in mussel foot proteins. A search on Google Scholar for mussel foot protein yields nearly 23,000 citations.
Interest around this “king of cling” dates back to the late 1960s when researchers studied byssal thread formation and particularly the attachment strength of the mussel foot to surfaces.
In 1981, biologists J. Herbert Waite and Marvin L. Tanzer shed light on an amino acid found in the byssal threads of the foot of the blue marine mussel, Mytilus edulis, as well as the contributions of an acid-soluble protein in the foot’s adhesive properties.
Fast forward 26 years—scientists from Idaho National Laboratory published research in Marine Biotechnology after their study of adhesive proteins identified in Mytilus edulis. The authors, Heather G. Silverman and Francisco F. Roberto, addressed the goal of developing “water-impervious, ecologically safe” adhesives similar to mussel adhesive proteins that “can bind materials ranging from glass, plastics, metals, and wood to materials, such as bone or teeth, biological organisms, and other chemicals or molecules.”
And Foston’s colleague and co-investigator on the EMSL project, Fuzhong Zhang, has proven that synthetic proteins created with engineered bacteria can be more adhesive than natural mussel foot proteins.
“He’s (Zhang) taken segments of muscle foot proteins and added them to segments of proteins found in spider silk fibers and amyloid fibrils,” Foston says. “Using genetic engineering, we have precise control over protein sequence and molecular weight, thus control over the ultimate properties of the material.”
In this EMSL project, “Characterization of Novel Synthetic Biological Materials,” the team is looking to connect the scientific understanding of the sequence of recombinant proteins and how those proteins fit together in the hydrogel with hydrogel properties. Recombinant proteins are created by combining genetic material from two different sources.
Nature-inspired materials in synthetic biology
When one thinks of research involving mussel feet, the first image that comes to mind might be scientists in full-length white lab coats huddled around commercial-grade sinks filled with mounds of shiny, deep blue shelled crustaceans.
The reality? Foston and his team won’t be stepping foot near the ocean nor will these creatures be sitting within the walls of their work space.
“In these types of applications, we’re using either natural proteins for this kind of work or we’re modifying them,” Foston explains. “So we’re actually not going to go out and harvest muscles for this. We’re going to understand what proteins the mussel makes, and then we’re going to ask a bacteria like E.coli to make it for us the same way they might do for insulin.”
At one time, this research may have been done with insulin from pig pancreas. But now, genetically engineered microbes make insulin.
“What we know is nature has done something that’s really, really cool,” says Foston. “Let’s learn from that. And then let’s also use the machinery of nature.”
In this particular project, Foston and the team will make proteins that are chimeras of silk mussel foot proteins with amyloids, which are aggregates of proteins, and silks. The amyloid segments are used to replicate the crystalline domains in a synthetic polymer like nylon. The silk segment represents amorphous domains, and the mussel foot protein segments add overall adhesion. Through synthetic biology, scientists can change the length and sequence of the amyloid or silk segments or the order in which they appear in the protein.
“So what we’re looking at is all of these effects and seeing how they change, say the mechanical strength of the hydrogel and its adhesion to various substrates,” Foston says.
Repairing tendons, boats
If scientists are successful with creating a hydrogel for industrial or medical applications, what would that look like?
Tendon-to-bone repair is a challenging surgical task because it has to connect biomaterials with completely different properties. Foston and his team believe a hydrogel created with specific properties could improve surgical outcomes, particularly for surgeries involving repair of tendon to bone.
“Sutures end up putting stress concentrations on the tendons,” Foston explains. “They have to anchor tendon to bone in some kind of way, requiring sutures that puncture the tendon. These stress concentrations lead to repair ruptures by the tendon pulling through the sutures. So technologies such as adhesive films along with sutures have been proposed to distribute stresses over a larger area and lead to better outcomes.”
To achieve this, Foston says the hydrogel needs the proper combination of biocompatibility, shear strength, shear modulus, and adhesion strength. This has to be balanced with a design that takes structure and property relationships into account.
For boat repair, a hydrogel based on mussel foot proteins could be a step up from available options. There is a need for adhesives that can patch cracks and damage, while being strong enough to be deployed underwater.
“These kinds of muscle foot proteins have shown to be really efficient in sticking to their various types of surfaces,” Foston says.
Studying recombinant proteins with EMSL resources
The vision for Foston’s EMSL research project is to form the synthetic protein into the hydrogel because it would be used as surgical adhesive or to repair watercraft. To achieve these results, Foston and his team will access a treasure trove of EMSL instrumentation. These include nuclear magnetic resonance (NMR), cryogenic transmission electron microscopy (cryoTEM), Raman spectroscopy, confocal microscopy, Fourier transform ion cyclotron resonance mass spectrometry, and the Tahoma computing capabilities.
EMSL’s NMR will be used to look at cross-links among proteins in the hydrogel, based on modifications the research team has made in mussel foot protein sequences. CryoTEM will help the team quantify the effect of amyloid crystals and muscle foot protein cross-links on the protein self-assembly. Mass spectrometry and Raman spectroscopy will provide high-spatial-resolution images that might help the researchers understand the chemical or molecular segregation that might occur as the hydrogel forms.
These resources and the experts at EMSL, Foston says, will assist with evaluating how polymers fit together with the hydrogel and how these components affect strength, toughness, and adhesion.
“The people that are there are some of the best in the world at what they do, so being able to tap into that is useful,” remarks Foston.