Bonding Over Science Episode 3: The Muscle of Mussel Feet
EMSL user Marcus Foston explains the muscle of mussel feet and how they could be used for hydrogels.
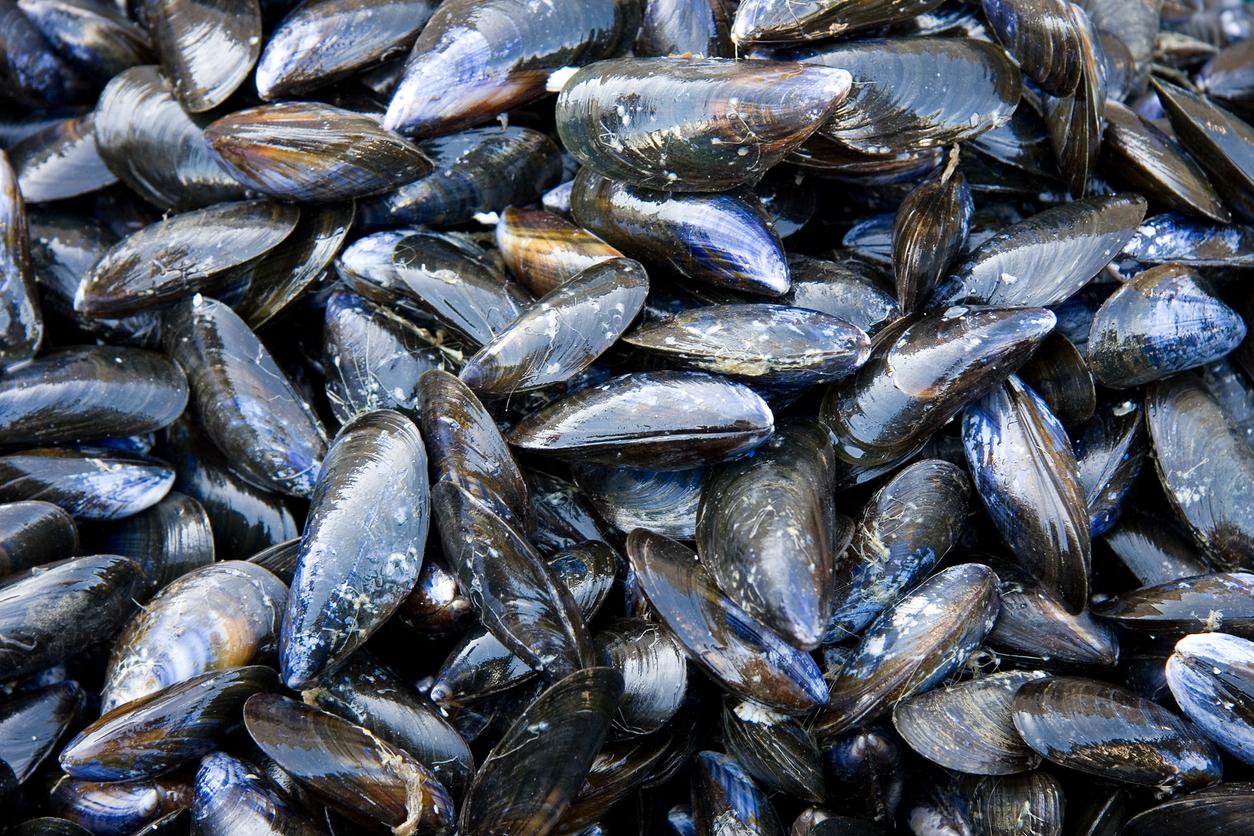
EMSL's Bonding Over Science podcast latest episode discusses mussel feet and how researchers are using synthetic biology to mimic the mussel foot protein. (Photo courtesy of iStock | Rixipix)
On episode three of Bonding Over Science, host Dawn Stringer talks to Environmental Molecular Sciences Laboratory (EMSL) user Marcus Foston, who is using synthetic biology to create an underwater hydrogel that mimics the strength of mussel foot proteins. Listen to Foston explain how this hydrogel’s powerful adhesive could replace surgical sutures and fix cracked boats. Read more in the web feature about mussel feet research.
(Animation by Stephanie King | Pacific Northwest National Laboratory)
(Music by: Bensound / License code: LOGRYFTIJJATJOSY)
Transcript:
[start]
<<music>>
Dawn Stringer: What is a mussel? No I’m not talking about your biceps. I’m talking about the little sea creatures in your favorite seafood fettucine.
Believe it or not, they’re good for a lot more than fine dining. These organisms have a strong organ called a foot that gives them the ability to move around. If you’re picturing Marcel the Shell with shoes on like I am, A-plus for creativity, but not quite the right idea. Researchers have learned these mussel feet have uses that range from boat repair to surgical use, and it’s a great example of taking advantage of material science to replace other products that create waste impacting our planet.
I’m Dawn Stringer, and this is Bonding Over Science.
<<music>>
Dawn Stringer: For today’s episode, I spoke with an EMSL user who is finding ways to replace various materials used in society with something already created by nature.
Marcus Foston: My name's Marcus Foston, I'm an associate professor here in Washington University in Saint Louis in the Energy, Environmental and Chemical Engineering Department. I've been here for about 10 years now, and my lab primarily focuses on thinking about ways in which we can replace petroleum for the primary source of our fuels, chemicals, and materials. We focus a lot on the conversion of lignin cellulosic biomass into chemicals and materials, but we've also started in this field of starting to think about working with some of our colleagues that work in synthetic biology, who are thinking about using biology as a framework to replace some of our materials as well. So this is what this project is really related to.
Dawn Stringer: So first and foremost, why would you want to replace these petroleum products?
Marcus Foston: You know, the motivation for a lot of this work is really related to the idea that currently right now, plastics are a significant problem, right? They're ubiquitous in our society, they are really responsible for a lot of the quality of life and ease of life that we see in today's world. Almost every in-use product has some amount of petroleum-based plastic in it.
But plastic waste is a grand challenge for our society and for our globe. I think we produce something like 150 million tons a year of plastic waste, and it literally pollutes almost every bit of soil and water on the planet to some degree. There's a lot of work that's been done looking at nanoplastics. Eventually, the mechanical action and chemical degradation of plastics in the environment, even though they persist for a really long time, they cause them to fragment into smaller and smaller pieces. And there's evidence that these nanoplastics are everywhere in the oceans, and if they're everywhere in the oceans, they’re everywhere in our body. There's information that suggests that we're on track to have more plastic waste in the ocean by mass than fish by the year 2050. So looking at ways of addressing this problem are going to be super critical.
Dawn Stringer: Right. And 2050, that's really not that far away.
Marcus Foston: Right. Yes. I mean, that's what's really scary about some of these numbers, right? It sounds like just a tremendous amount of plastic waste. And then, of course, the CO2 that's associated not only with the materials themselves, but then the production of these materials. And so, you know, as people are getting better at producing let’s say biofuels for things like large transportation—like airplanes, ships—as we become better at making renewable electrons, we're going to have to also be able to produce our materials for something other than petroleum as well.
Dawn Stringer: So we can see how this is so important to the atmosphere, our bodies, the world. Tell me how you got involved in this research that we're going to talk about today and what it is.
Marcus Foston: I have a colleague here, Fuzhong Zhang, who is in the field of synthetic biology, which is essentially how do we reprogram microbes to make materials or make chemicals that we want them to make, right? And so we've been able to take these natural pathways and we start to think about, well, how can we change those? And now that we know about DNA and how to do genetic engineering, and the Department of Energy and a number of other places have been really, really pushing genetic engineering, synthetic biology for the production of fuels.
At first, biofuels and chemicals. And now the next evolution of that is materials. My colleague, plus a few other people who are also in our department—we have a core expertise in synthetic biology—started thinking about these. How can we use biology, how can we use synthetic biology to produce materials? My background is in materials science, and so I've come from the place of thinking about originally about polymers, you know, plastics that are derived from petroleum, and using organic chemistry methods.
And I started to apply that to thinking about biomass, so lignocellulosics about plant material. And so it was just a natural evolution, and once I saw them working on this and say, “Hey, maybe I can lend my expertise from the other side of things with respect to understanding polymer science and engineering, maybe I can help you with your project.”
And so that's kind of how I got that really interested in the project. And of course, it's just a really cool idea, right?
Dawn Stringer: It is! And I have to admit, when I first saw the term mussel feet, Marcel the Shell with Shoes On is the first thing that popped in my head. If you haven't seen it, you should definitely Google it. It's really cute. But what actually is a mussel and how are you using it?
Marcus Foston: The mussels are very similar to what the cartoon is describing, right? It’s these shell crustacean organisms. They are filter feeders. They need to position themselves where they are in kind of like moving water, and so they often have, they have a strategy, you know, whether it's rocks or a side of a dock or even ships, they have these mechanisms that allow them to stick to surfaces.
So they secrete essentially a very natural glue. And so people have studied this for a very, very long time. The idea is that if we can understand how these mussels or other crustaceans are sticking themselves to surfaces then they can produce better adhesives. That's the idea. So people have studied this for a long time, and in particular, the mussels, mussels have a protein that they will produce that essentially anchor them to let’s say the side of a surface.
And so this protein, this protein assembly, is actually called the mussel foot. And so that's where that term comes from, it's a mussel foot protein. It's actually quite a large kind of like protein assembly. And they, you know, kind of start to understand what the sequence of these proteins are and they start to sort out some of the function of the proteins.
And a lot of what they've been able to discern is related to the fact that these proteins have these tyrosine amino acids that get oxidized into dopamine. And so there's a vast amount of organic chemistry and polymer chemistry that tries to mimic these types of protein-based materials to produce underwater adhesives. So instead of trying to use organic chemistry and starting with carbon that is coming from petroleum, my colleagues, and a number of other people before them as well, thought, “Well, why can't we get E.coli or some other kind of microbial system that we're really good at engineering, why can't we get them to produce this mussel foot protein for us instead of trying to make some synthetic version of that?” And so that's kind of like where the idea, at least of the muscle foot part, comes in.
Dawn Stringer: So it sounds like instead of going and harvesting all these mussels, people might be concerned about the population, it sounds like you're mimicking that biology to use to our benefit.
Marcus Foston: Yeah. It's not clear to me that you would be able to cleanly extract that protein out and still get the kind of material properties that you might want to see as far as adhesive properties go. I mean, this is a growing field now where we can look towards nature, and nature has, through millions of years of evolution, often landed on these various materials that are really, really functional.
They work really, really well. Instead of trying to redesign what nature has figured out, the idea is like, okay, well, let's look towards nature. Just use that as a starting point because evolutionary pressures are always going to dominate the development of these materials. And so, like, evolution is not necessarily making materials for human purposes, it’s making it for whatever organism is using it.
Maybe we can get around that particular aspect of evolution and find something that is maybe has that kind of mechanical properties or material properties that we want. So it has the proper type of adhesion that sticks to materials that maybe aren't normally found in nature or in the case here that has higher strength and toughness properties than you might normally find.
So we start with these mussel foot proteins that we know have the capability to have high adhesion onto a wide range of materials. And we start with these proteins that have been studied for some time. And so the kind of chemical and molecular phenomena that lead to adhesion has somewhat been uncovered as well. So we know, for example, pi stacking, hydrogen bonding, all these things play a really key role in the way these poly-dopas will stick to surfaces.
And so then the question is, can we splice in other types of materials? Can we put in silks, can we put in amyloids to kind of make the material have enhanced mechanical properties? So for example, we have the NSF, National Science Foundation, grant that thinks about potentially using these same types of materials as surgical adhesives as well.
So you can think of a number of applications where, you know, you have this wet environment, you need to have high, you need still need to have kind of great adhesion. But maybe the place where you're doing sutures was actually on a joint or a knee and you need something that has a significantly higher amount of mechanical stiffness or ability to stretch than the muscle foot protein would have by itself.
And so by doing these kind of modifications, splicing in other types of sequences, the hope is that we can develop them, those additional properties.
Dawn Stringer: Now with that specific application, what is currently being used for this purpose, and why could this be better?
Marcus Foston: Typically, let’s say you get some type of ligament replacement, you'll have a bone and they have to use sutures and actually kind of stitch the ligament onto the bone itself, right? And drill holes in the bone and they’ll stitch it. And they’d still have to do that even in a scenario where you have this adhesive, but these sutures put a lot of point stress on the ligament.
And so often when the repair actually does fail is at these point sources of stress. And so the idea is that you not only having sutures, but then you would want a strong and tough material, right? So something that had the right kind of mechanical properties that it would mimic, that something that would go in the body.
It would need to be biocompatible, right? It would need to have all of these properties and then you would want something that would actually have an adhesive component to it as well. And so it's essentially kind of like putting a patch on holes and a suture that you put into whatever you just kind of stitched up, because that patch, this stress, they will spread that stress out over a larger area.
And so you have less chances of failure. But again, the real key is the material design is really hard to get any kind of artificial material to kind of fit all of the criteria that we just talked about, even something that people have been using for a long time, like Super Glue, you don't really want to put it inside of the body itself, right? And so this is kind of like the perfect application, kind of initial application, the proof of concept that these types of materials have significant value.
Dawn Stringer: Do we know how long this has been studied and how long we can wait to see this actually be applied?
Marcus Foston: Much like many technologies, I think people have been thinking about how we might use genetic engineering to make materials for quite some time, in particular silks and those in mussel foot proteins. These are proteins that people have been targeting for a long time. And even people are thinking, have been thinking about how to splice them together. The angle that we've been taking is to then try to overlay a deeper understanding of mechanics, of polymer science and engineering, even deploying some aspects of computer science to help accelerate this material discovery and understanding systems.
And, you know, there are some products out on the market that probably, now, that depend somewhat on kind of recombinant technologies to make materials, but the kinds of materials that we're developing right now, they're probably, you know, years away from translation. But from what I understand, that's also usually a pretty extensive and lengthy process as well.
Dawn Stringer: What role does EMSL play in this research?
Marcus Foston: In polymer systems, often the sequence or protein-based systems, the protein sequence determines the way in which the protein or the polymer wants to fold, the structure it wants to take in whatever hydrogel or fiber that we ultimately end up producing. EMSL has a really advanced suite of characterization techniques that are really ideal for looking at not only the molecular structure of our protein, but its ultra-structure, right? To understand how sequence and processing has affected that structure.
And then ultimately, we have the capability of measuring properties. So a lot of times what we're trying to do now, is the goal is to try to find these relationships among sequence structure and then the ultimate property that we see from the fiber or hydrogel.
Dawn Stringer: You mentioned the capabilities at EMSL, what capabilities in particular have you utilized for the mussel feet research?
Marcus Foston: One of the things that we're really interested in is using the cryo electron microscopy capabilities at EMSL. You know, these proteins will form a particular structure depending on the sequence of the processing we put it through, and that particular technique combined with other things like X-ray and neutron scattering that we use let’s say at another DOE facility like Oak Ridge, could be really, really useful.
We also are really interested in applying solid-state NMR to try to understand, again, the kind of structure that these polymer systems adopt, and so EMSL has a number of NMR instruments that are capable of helping us answer this question as well as the kind of specialized expertise that it really takes to value-rize NMR.
And then finally, we're really interested in investigating chemical and compositional distributions. We're understanding whether or not these various segments of these proteins tend to face, segregate, and orient with one another in different areas, depending on how we put them together and process them. And so there's a range of imaging modalities. They're rare-ish techniques that you won't see in a lot of universities or even user facilities, that we are also tapping into at EMSL.
Dawn Stringer: What are some other aspects that you like about working with EMSL?
Marcus Foston: I, you know, kind of consider myself a little bit of an analytical geek. I love to kind of try to think about ways of using these kind of sophisticated techniques to understand something unique about the system and then really figure out how you place that into an engineering context to make decisions about the way you design a material, how you produce it. Often, these techniques, the development of them are very sophisticated, so they often kind of get put into a small little sphere of people who either understand them and so they don't get applied in ways that help us, you know, really answer really tough engineering questions.
And so that's kind of been like kind of a hobby of mine or a career hobby of mine over the last couple of years. How do we do that? Being able to interact with a place that has so much unique capability and has so much expertise, that is all designed around supporting users, that the facility is set up that way, the management is set up that way, it's really nice to be able to interact.
Dawn Stringer: In the future, what do you hope to see with your research?
Marcus Foston: What I really think as we move forward, material manufacturing will move towards a stage where biology becomes really important, so the biologicalization of material science and manufacturing, essentially, and then also the ability to be able to make these small changes in sequence. So for organic chemistry to change, to make well-defined polymers, and to change a single monomer unit in the polymer strand, it can be quite difficult to do that.
We can do that here with a small tweak of DNA. I mean, so we can go through vast permutations of a particular material, and so now it's just overcoming the challenge of how do you then produce enough material so that you can do material testing and how do you do high-throughput material testing? And these aren’t straightforward questions, but these are things that people are thinking about.
And so once you do, you know, once you do those kinds of things, then it really opens up the power of AI and all of these other things that we've been talking about, not particularly in this podcast, but that people in our field have been talking about leveraging, it really opens up the capabilities to do that. So that's what I'm really excited about seeing come from this particular set of projects we're working on now.
Dawn Stringer: With so much going on, where can our listeners keep up with your work?
Marcus Foston: Yeah, so if you just come to the Washington University website, our school is the McKelvey School of Engineering. Again, I'm in the Energy, Environmental and Chemical Engineering Department. If you come to our website, you look up our faculty and you look up my name, Marcus Foston, you should be able to find my last website, and we'll also be spinning up a center that is really focused on developing these technologies. And it'll be the Center for Manufacturing Synthetic Biological Materials. There should be a link to that coming up soon.
Dawn Stringer: What do you think is important that you want to leave our listeners with?
Marcus Foston: Yeah, I mean, as a kind of closing remark, another aspect of the center and another area that I am particularly interested in, that we'll be thinking about, is this intersection between, you know, making technical advances and thinking about ways in which technology is adopted. You can come up with the best technology out there, and there's always an additional component to that technology and it’s humans. And so understanding the ways in which stakeholders, various stakeholders, whether it be the producers of goods, so we're talking about industry here, whether it be consumers, how they think about these technologies, how we as researchers and engineers talk to these various stakeholders, how we try to understand not only kind of the technological need that they have, but the kind of like psychological and sociological inputs that go into these technologies.
I think all of this is going to be a really important thing. I think some advances that we've seen come out in the last few years that haven't been adopted, the ways you might expect them to be adopted, I think it shows the need for this kind of understanding. And so, you know, for me, for my students, I think having a broader understanding of humans is going to be super important for the future of kind of like the way in which we develop technologies that affect society.
And hopefully from the work that we do here, we start to make a little bit of progress in understanding those things. But more importantly, we start training the next generation of engineers and scientists to better appreciate those factors.
Dawn Stringer: Well, Marcus, thank you so much for joining me today and being on Bonding Over Science.
Marcus Foston: Well, I really appreciate your time as well. It's really fun to get to talk about your research, and I actually really appreciate EMSL, their mission and how we've been able to collaborate in the past.
<<music>>
Dawn Stringer: Since nature took the lead on putting the muscle in mussel, researchers like Marcus are stepping in to learn from their biology for our benefit. For more information on this project, you can read the full article at emsl.pnnl.gov, and don’t forget to check out our social media platforms for all new things coming from EMSL!
<<music>>
Dawn Stringer: EMSL is a Department of Energy, Office of Science national user facility that accelerates scientific discovery and pioneers new capabilities to understand biological and environmental processes across temporal and spatial scales. EMSL leads the scientific community toward a predictive understanding of complex biological and environmental systems to enable sustainable solutions to the nation’s energy and environmental challenges. If you’re interested in working with EMSL, learn more at emsl.pnnl.gov, that’s E-M-S-L-DOT-P-N-N-L-DOT-G-O-V.
[end]