Functional and Systems Biology
Environmental Transformations and Interactions
Investing from Within: Biology Gets a Boost | Part 2 of a 3-Part Series
Imaging roots, chasing protein functions, and more benefit from supporting new internal science and capability development
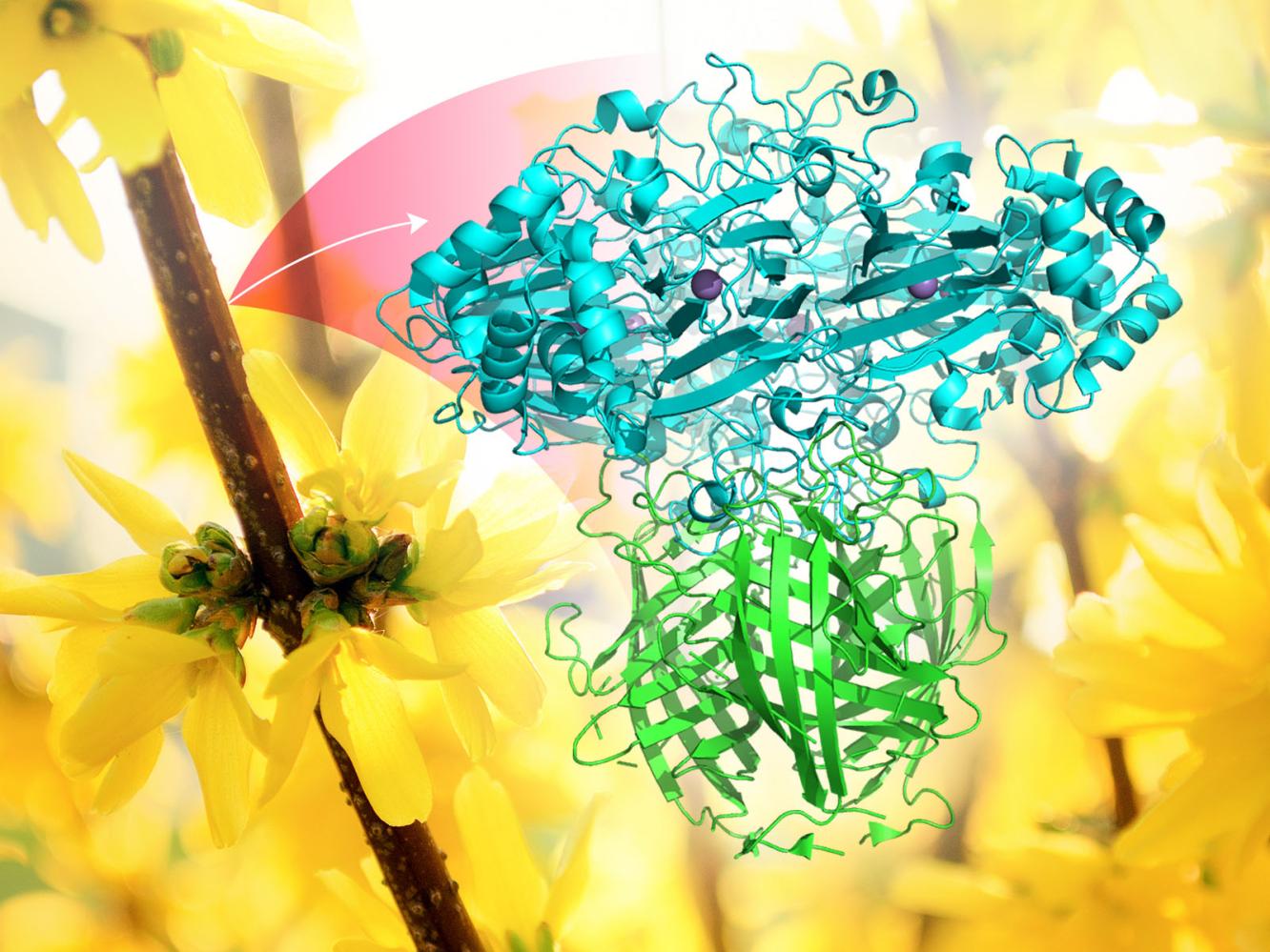
Before long, Laboratory Fellow Emeritus Christer Jansson and a few colleagues will have three-dimensional images of metabolic and microbial interactions in the root system of a hardy grass, sorghum, that has promise as a biofuel crop.
In another corner of the user facility, EMSL proteomics chemist Mowei Zhou is close to identifying obscure proteins that control the production of lignin, a foundational plant polymer.
Irina Novikova, an EMSL expert in biological nanoimaging, is working on cell-free protein synthesis technologies that yield high-purity samples for analysis.
Jansson, Zhou, and Novikova are just three of the many Functional and Systems Biology scientists who received internal facilities research funding from EMSL in the last two years to pursue novel ideas.
This internal funding resource at EMSL, about $5 million a year, is designed to acknowledge and accelerate targeted research by staffers in all areas of inquiry at EMSL. Unlike traditional Laboratory Directed Research and Development (LDRD) internal funding provided by Pacific Northwest National Laboratory, such internal EMSL investments are not intended as a lead-up to competition for external funding. Instead, they are intended to build science and capability in support of the EMSL User Program.
“At the highest level,” said EMSL Chief Science Officer Justin Teeguarden, “these are opportunities for staff to be continually innovating―thinking of ideas and moving them forward.”
Tiers of Internal Funding
Teeguarden, a biomedical scientist who started his new role as EMSL’s Chief Science Officer at EMSL in October 2020, arrived just in time to see a new list of internally funded projects get underway. A call for proposals went out in July 2020; awards were announced in October.
This kind of funding is available not only in the realm of biology, but also in EMSL’s two other formal areas of inquiry: Environmental Transformations and Interactions (where internal funding was outlined in Part 1 of this 3-part feature) and Computing Analytics and Modeling.
Internal funding is layered, based on a system started in fiscal year 2020. Tier 1 funding is for projects running a year or less and is open for proposals throughout the year. Tier 2 projects get a single year. Tier 3 projects get two years of support.
In all cases, however, funded projects “are aligned with where EMSL wants to go,” said Teeguarden. “We’ve got a strategic roadmap.”
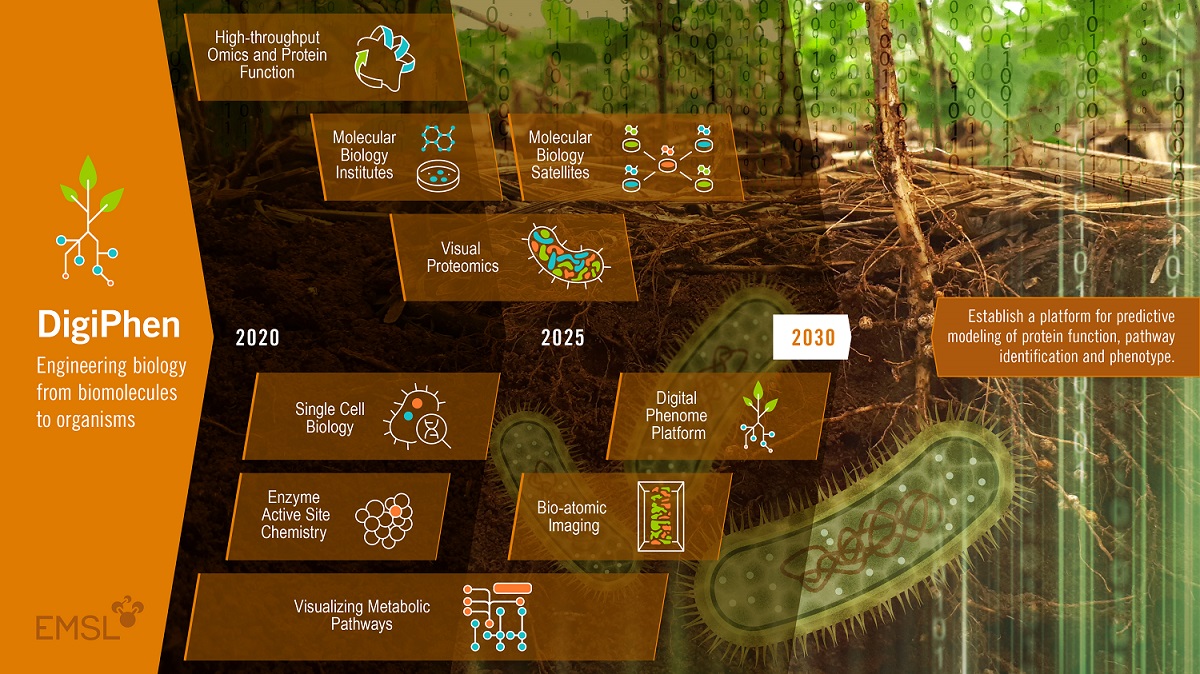
Fundamental Mission First
EMSL’s 2018 strategic document outlines goals that pivot on its fundamental mission, in concert with that of the U.S. Department of Energy (DOE): to better understand biological and environmental processes across a wide range of temporal and spatial scales. That is, from the scale of molecules activated on the surface of plant roots, as in Jansson’s research, to ecosystem-size processes that determine the fate of watersheds.
In part, such understanding is in concert with one aim of DOE’s Biological and Environmental Research (BER) program: to develop a predictive understanding of complex systems in order to engineer them in support of U.S. energy security and environmental sustainability.
For instance, consider Zhou’s project on lignin-related proteins. Lignin is one of three main polymers in plant systems. (The others are cellulose and hemicellulose.) Its role is to lend rigidity to plant-cell walls, which makes wood and bark so tough and resistant to digestion.
The more scientists know about the proteins which synthesize lignin, the better they can overcome lignin’s tough structure to access the plant’s energy-rich glucose chains bound up in cellulose. And the better scientists can use lignin as a carbon source for biofuels and bioproducts.
Visions for 2030
Proposals for internal funding are also examined through the lens of EMSL’s vision of its science by the year 2030, which is like a booster rocket for BER’s predictive mission.
Part of that 10-year vision for EMSL’s biology research is an emerging platform called DigiPhen (short for Digital Phenome). A phenotype is an observable set of characteristics generated when an organism reacts with its environment. Genes and the proteins they encode and their interactions with the environment are associated with that phenotype.
Proteins control a cell’s structure, function, and regulation―yet many have not been functionally identified. DigiPhen will speed the modeling of proteins and therefore our predictive understanding of them.
Trends in internal funding at EMSL show that “one major area is structural biology,” said Scott Baker, a fungal biotechnologist who leads EMSL’s Functional and Systems Biology track and oversees the DigiPhen effort. “It’s a big emphasis for us.”
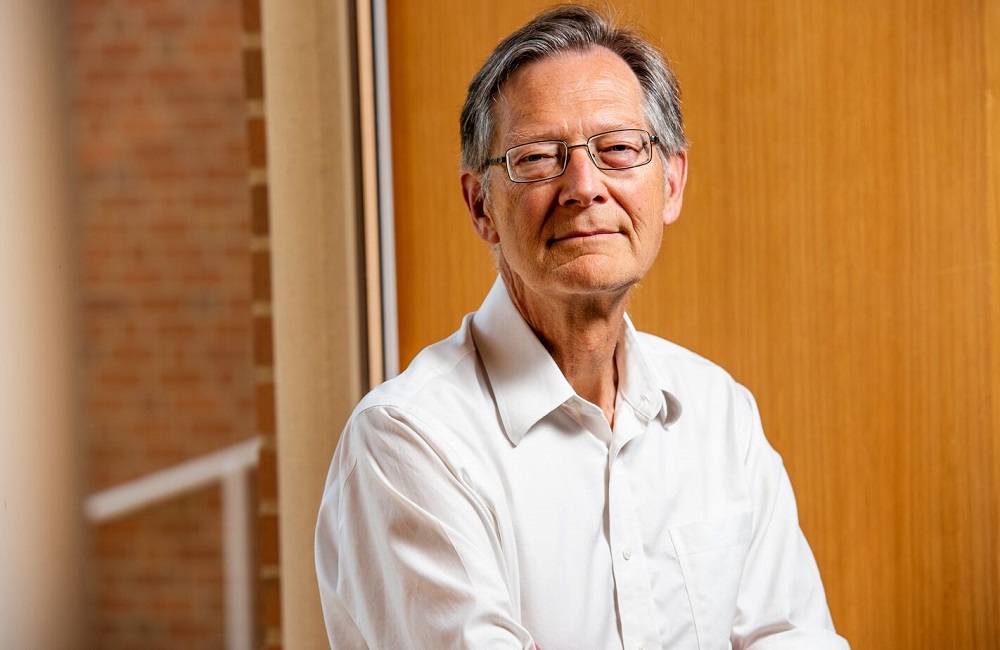
Jansson: Metabolites in the Rhizosphere
Christer Jansson, and a team of fellow researchers, are investigating intercellular molecular interactions in the rhizosphere. The research team is focused on grass systems that have promise as bioenergy systems.
One of those grasses is sorghum, “a wonderful crop,” said Jansson. It is high-yielding, drought-resistant, and in warm and dry conditions will out-compete other plants. Fortunately for researchers, sorghum also has a simple, well-studied genome, which has been fully sequenced.
With that genomic head start, he said, “we have a lot of collaborative cooperation,” including with the Joint Genome Institute in California, which (like EMSL) is a DOE user facility.
With EMSL botanist Pubudu Handakumbura as the technical lead, and together with EMSL Earth scientist Albert Rivas-Ubach, the team is investigating what kind of molecules are active within the root system of sorghum.
They ask: What is being exuded from the roots? What controls microbe-soil-metabolite interactions? How does any of that confer drought tolerance? Are there “hot spots” where metabolite activity is especially high?
Microbes of Interest
The project was funded in FY 2018 through EMSL Facilities Research investments. That initial work wrapped up in the fall of 2020. But it’s being continued as part of the DOE’s Persistence Control Science Focus Area (SFA), led by Pacific Northwest National Laboratory (PNNL).
In a laboratory setting, the team marks microbes of interest with genomic tags that shine green or red with a 3D study space. Researchers have captured images of root-colonizing microbes in a root system about 7 centimeters (2.7 inches) long. They have also analyzed what metabolites are present. (A paper summarizing the first phase of the process is in process.)
There will soon be video images that showcase this approach, said Jansson, including a 3D rotating map that indicates where tagged metabolites and microbes are located within the rhizosphere. That is coming by way of PNNL’s National Visualization and Analytics Center.
Jansson credits many others in the sorghum work. PNNL microbial ecologist Janet Jansson initially envisioned the project and has access to the software that makes it possible to map the root system. EMSL Senior Research Scientist Tamas Varga and his group helped generate and process images from x-ray computed tomography. Earth scientist Tom Wietsma and his team constructed necessary hardware
The Jansson team created a sand-like laboratory soil substitute to overcome one difficulty of taking pictures of a 3D underground realm: Natural soil has a density nearly the same as root systems, making imaging contrast between the two difficult.
A computer-aided 3D image of the sorghum root system was the “first step,” said Jansson. “Now we’re working on (imaging) the microbial and metabolite systems to see how the plant functions underground. We’re starting to understand what controls the colonization of microbes at the roots.”
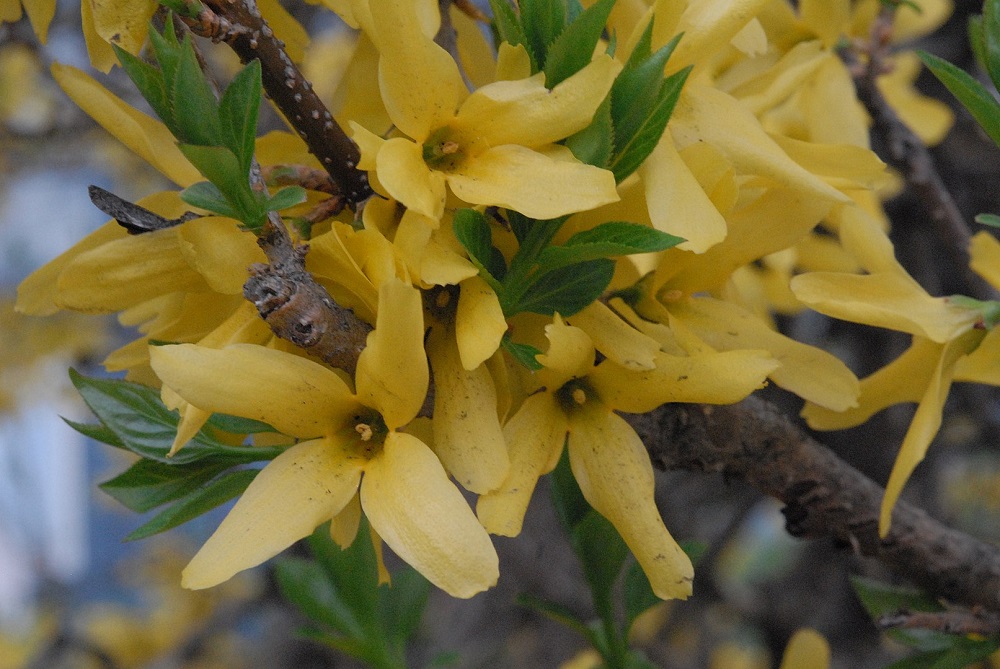
Identifying Useful Proteins
Jansson deals with sorghum, a candidate bioenergy grass with a well-defined and fully sequenced genome.
Mowei Zhou, on the other hand, is dealing with border forsythia (more formally Forsythia x intermedia), an ornamental shrub that produces bright yellow flowers and can grow 12 feet high. It lacks a complete genome, but it is the botanical platform for researchers in the Norman Lewis group at Washington State University, who are using Forsythia x intermedia to investigate dirigent proteins (DPs).
Linked to the formation of the lignin, DPs evolved eons ago when plants transitioned from aquatic to terrestrial environments. They are believed to affect lignin structure during the early steps of biosynthesis. That makes DPs intensely interesting to biofuel researchers looking for ways to break down plant-cell walls.
Lewis and his team employ biophysical technologies at EMSL to unveil the architecture of plant protein complexes that contain DPs. To date, researchers have identified fewer than 10 percent of the large multi-gene families related to these important proteins.
Zhou’s project, still supported by EMSL internal funding, began in FY 2019, when he took receipt of samples Lewis, an EMSL user, submitted for analysis.
“The intent is to identify other proteins that work directly together with DPs,” which offer vital clues for how to control lignin biosynthesis, said Zhou.
A Hunt for Enzyme Targets
Because there is no sequenced genome, Zhou uses mass spectrometry to look for homologs―proteins with a shared ancestry―in plants that have well known genomes.
“The challenge is to isolate DPs from other, more well-studied plants with genomes,” said Zhou, who has a related paper underway on the data integration required. “That’s really hard.”
With the help of multiple instruments at EMSL, including time-of-flight and Orbitrap mass spectrometers, “we have identified several enzymes we were expecting to see―and a few we were not,” he said. “We’re working to capture more. Ideally, we want to be able to identify every interacting protein to see a high-resolution structure of the whole enzyme machinery.”
With a degree of control over a suite of dirigent proteins, he added, “we can tune the lignin structure to make it harder or softer.”
Practicality is the ultimate aim.
“Once we have identified the protein structure and function,” said Zhou, “we can begin to engineer it―to make it easier to degrade and break down (proteins) faster for the biofuels mission.”
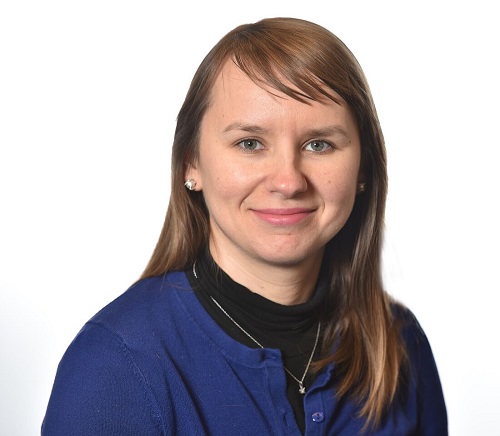
Novikova: Pursuing Glycoproteins
Glycoproteins are proteins enzymatically modified with glycans—various sugar molecules—chain-linked in a diverse branching architecture. This protein-modification process is called glycosylation. It is the major (and most frequent) modification detected in plants, animals, and fungi.
Glycoproteins are abundant in nature. About 10% of all proteins in the human proteome are glycoproteins. And the dirigent proteins Zhou is working on―the ones critical for lignin synthesis―are glycoproteins.
That abundance makes it “crucial to know more about the glycosylation process,” said EMSL chemist Irina Novikova, a specialist in getting nano-scale images of chemical and biological processes. “We want to understand what each attachment does to (target) proteins. We need to know what these glycans do.”
In 2019, Novikova began work on a one-year internally funded capability development project designed to create a toolkit to analyze glycosylation in plants―a first step before branching out into other life forms. EMSL recently refunded the project for an additional year.
A glycosylation toolkit, within the confines of a test tube, enables researchers to introduce one glycan at a time to see what that glycan does to a target protein. So far, such toolkits are only available for bacterial systems, which are far less complex than plants.
“No one has a toolkit suitable for plant systems,” said Novikova. “This is a unique opportunity to develop something similar to bacterial toolkits. At EMSL, we have all the right tools and expertise.”
A Path to Modifying Proteins
The branching, composition, and number of glycan attachments per protein can be highly variable, depending on the organism, cell type, and many other factors. This variability determines how a protein will fulfil one functional role or another. However, the diversity of glycans results in very heterogenous samples and makes them extremely hard to study.
Glycosylation toolkits make it possible for scientists to create artificial enzymes. They can also use glycosylation toolkits to “modify the nature of any protein you want,” said Novikova, who wants to expand her work “beyond plants, into the fungal world.”
Toolkit technology can also help characterize protein structure and function. That’s one of the main goals in EMSL’s biology research realm and is connected to DigiPhen.
Novikova is more than halfway―60%, she estimates―to achieving a glycosylation toolkit for plants. So far, her system can express proteins and reconstitute them. And she has a glycan precursor already in hand.
The toolkit will “be beneficial to many EMSL users,” said Novikova. “I just need to demonstrate I can do it.”
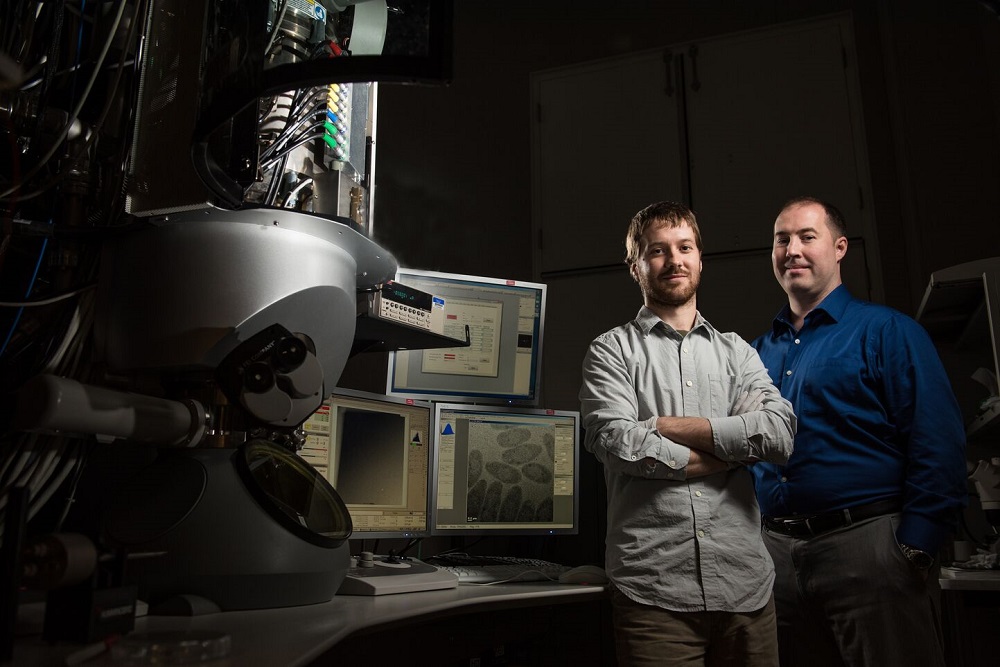
Evans: A Cryo-EM Triumph
In the summer of 2019, scientist James Evans utilized $2.9 million in EMSL funding for a state-of-the art Cryo-TEM―one half of the sum needed to purchase the microscope and to support operational funding in 2021 and 2022. The other half was co-funded by BER’s Biological and Systems Science Division.
Cryo-EM (the name for the technique, not the device) allows researchers to determine the structure of flash-frozen biomolecules at near-atomic scales. At EMSL, technicians installed the microscope in the summer of 2020 and mounted its first samples in October. Evans team members Trevor Moser and Amar Parvate are the Cryo-TEM’s day-to-day operators and interact with EMSL users. Both are recently hired EMSL scientists.
Parvate spends part of his time on a project with long-time EMSL user Michelle O’Malley of the University of California, Santa Barbara. It involves cellulosomes, fungal enzymatic complexes that degrade woody plant matter with machine-like efficiency and reside in the gut of ruminant animals. With an eye to biomass processing, he is using a single-particle approach to investigate how these cellulosomes are assembled. Parvate hopes for insights into the function of the various subunits and how they interact.
Moser is working with another longtime EMSL user, Himadri Pakrasi of Washington University in St. Louis, who studies molecular machinery tied to solar energy. At EMSL, that means analyzing a cyanobacterial complex called Photosystem II. The hope, said Moser, is to acquire “a more complete view of the structure,” including the location and atomic details of some intriguing and little-studied accessory proteins.
Also part of the Cryo-EM team are EMSL’s Samantha Powell and Irina Novikova. Powell is working on linking Cryo-EM with native mass spectrometry. That’s a mass spec niche that allows protein samples to retain their native state during the gas phase of analysis, speeding the study of structure-function relationships.
The shared goal of the research team, he said, is to “create accessible workflows combining cell-free expression, native mass spectrometry and Cryo-EM to empower more holistic analysis of protein complexes.”
First, Internal Funding
As for Cryo-EM at EMSL, the origin of this dramatic improvement in capabilities came in fiscal year 2018 and 2019, when Evans oversaw a project funded internally by EMSL through a Facilities Research investment of the kind that gave Jansson’s sorghum work a boost.
“The EMSL-funded project helped create a pipeline for structural biological analysis,” said Evans.
It was a preemptory start on workflows for cell-free expression, whole-cell tomography, and single-particle analysis methods. In essence, he and his team were buying furniture for a house they hoped to buy.
Evans and his colleagues also leveraged the formation of the Pacific Northwest Center for Cryo-EM for which Evans is a co-investigator. The joint project with Oregon Health Sciences University is funded by the National Institutes of Health, which invested $50 million to purchase and operate five state-of-the-art microscopes. Several EMSL staffers help run the microscopes on site in Portland, Oregon. Other EMSL staff in Richland, Washington, manage the user portal, data access and computer resources.
“The combination of the EMSL project and the NIH center helped set the stage for us to secure additional investments in refreshing Cryo-TEM instrumentation at EMSL,” said Evans. “Things really fell into place.”
EMSL is funded by the DOE’s Biological and Environmental Research (BER) program in pursuit of earth systems work. The new Cryo-TEM was funded from the biological side of BER, said Evans. “It expands our user base and the program managers we interact with at DOE.”
The new Cryo-TEM, housed within EMSL’s Quiet Wing, replaced a nearly 30-year old liquid helium cryo-TEM instrument.
“We did not have state-of-the-art hardware, we did not have automation, we could not load multiple sample grids in one session, and we did not have remote operating capability,” said Evans of EMSL before the new Krios G3i Cryo-TEM arrived. “We were really in a fledgling position without that internal investment. There was no way for us to upgrade the old instrument. Now we have completely refreshed our Cryo-TEM capabilities and are applying them to advance EMSL user science.”
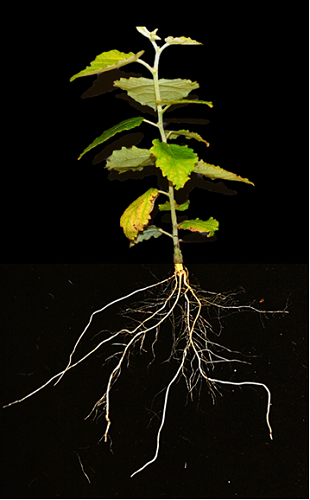
Ahkami: Carbon Allocation in Plants
Ahkami, the plant biologist who had an early role in Jansson’s 3D root-imaging work, was leading a two-year internally funded project of his own. It investigated the molecular networks that coordinate carbon allocation in plants. Active research wrapped up in the fall of 2020.
Ahkami and his project team have a manuscript in progress. It will provide insights into a complex gene regulatory network that has been little studied to date.
“Source-to-sink” carbon allocation refers to the transfer of energy from a source, the leaves where photosynthesis occurs, to a sink―a plant’s root system.
“We are trying to identify the key regulatory factors that control leaf-to-root sugar transport,” said Ahkami, the project’s principal investigator.
Working with him over the last two years have been EMSL’s Vimal Balasubramanian, Hugh Mitchell, Tanya Winkler, Dusan Velickovic, Chris Anderton, Pubudu Handakumbura, Albert Rivas Ubach, Jim Moran, and Christer Jansson. Ziyu Dai joined from PNNL’s Energy and Environment Directorate. Yuliya Farris and Robert Egbert joined from PNNL’s Biological Science Division.
The team is working with laboratory-based micro-cuttings of poplar plants grown in a gel medium. The cuttings are subjected to what Ahkami calls a new approach: measuring the fate of sugar transport as it is affected by auxin, a phytohormone that regulates plant growth.
More auxin spurs added root growth, said Ahkami, which causes new roots “to ask for more sugar.”
Based on adding more of the hormone, or withholding it, or blocking it with an inhibitor, the team subjects the resulting samples to gene expression analysis, stable isotope probing, and metabolomics. The aim is to map the genetic and metabolic regulatory network that controls sugar transport.
“That’s really novel for source-sink carbon allocation studies,” said Ahkami, and for the first time the process gives EMSL “a new platform for plant genome editing. Sometime soon, we can offer it to users.”
Internal funding brings with it an extra dose of freedom and creativity to research, he said. “You always have the right to change your approach. You can play with that. It’s a ticket for internal EMSL staff to make things happen from scratch.”